The detection and characterization of exoplanet atmospheres are perhaps the most exciting, frontier areas of exoplanet research, itself a nascent subfield of astrophysics. Now that is it well established that there are numerous earth-radius planets in the nearby Universe, and we have a rudimentary understanding of the statistical distribution of exo-earths and exoplanets in general (e.g. Fressin, 2013), the next frontier is to characterize planetary atmospheres – i.e. atmospheric chemical and molecular composition, particulate and haze content, heat transport & “weather” and the interplay of geophysics and atmospheric evolution.
Detecting O2 in the Atmosphere of Earth Twins
An important potential indicator of exoplanetary bioactivity is the presence of O2 in exoplanet atmospheres. As several authors have pointed out (e.g., Snellen et al., 2013; Rodler and Lopez-Morales, 2014), A-band of absorption features between 7600 and 7700Å are particularly useful spectral feature to search for during exoplanet transits.
While telluric foreground absorption feature would seem to constitute an insuperable impediment to detecting exoplanetary O2, the features are quite narrow and quite modest relative line of sight velocities between the Earth and an exoplanet are sufficient to Doppler shift the exoplanet feature out from under the telluric absorption, and the exoplanet signal becomes detectable if observed at high enough resolution (R ≳ 100,000).
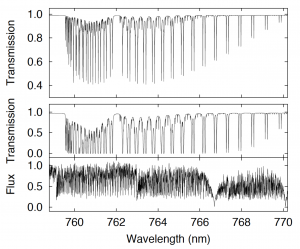
G-CLEF’s non-scrambled (NS) PRV mode will have the resolution to differentiate between Earth’s telluric lines and exoplanetary O2 signatures. Results of detailed analyses suggest that while fairly long observational campaigns may be required with the GMT to detect O2 in exoplanet atmospheres orbiting around G, K and early-type M stars, later-type M stars may be good targets for the search for bioactivity.
Type | Obs. Time (h) | Duty Cycle | Transits | Time (years) |
---|---|---|---|---|
G2V | 470 | 0.18 | 37 | 37 |
M1V | 133 | 0.86 | 33 | 35 |
M2V | 133 | 0.91 | 40 | 31 |
M3V | 130 | 0.94 | 44 | 28 |
M4V | 70 | 0.98 | 34 | 14 |
M5V | 79 | 0.98 | 53 | 12 |
M6V | 75 | 0.98 | 68 | 10 |
M7V | 61 | 0.98 | 78 | 8 |
M8V | 69 | 0.98 | 100 | 8 |
M9V | 67 | 0.98 | 154 | 7 |
Therefore, G-CLEF targets are expected to be dwarfs of class M3 to M8 within 20 pc of the Sun. This implies a magnitude range of MI =6.13 to 18. The bright end of this distribution is set by one of the brightest M dwarf visible from Las Campanas GJ 229. The faint end is set by the brightness of an M8 star at a distance of 20 pc.