Probing the Cosmic Dawn
The formation of the first luminous sources marks the start of a fundamental transition in the physical state of the early Universe. These first stars and galaxies produced ultraviolet radiation and heavy elements which reionized and chemically enriched the primordial material from which they were born. This cosmic dawn epoch lies at the frontier of modern observational astronomy and is one of the top priorities in astrophysical research in the forthcoming decade. Studies of the intergalactic medium (IGM) traced by absorption in the spectra of quasars and gamma-ray bursts (GRBs) at z ≥ 5 provide one of the key observational probes of the cosmic dawn and epoch of reionization (Fan et al., 2006, Totani et al., 2006, Mortlock, et al., 2011). Detailed studies of intergalactic absorption lines enable constraints on the ionization state of the IGM in the early Universe (Mesinger & Haiman, 2004, Mc Greer, Mesinger & Fan, 2011, Bolton, et al., 2011), as well as measurements of related quantities such as the IGM temperature (Becker, et al., 2011, Theuns, et al., 2002) and the abundances of heavy elements (Ryan-Weber et al., 2009, Simcoe, et al., 2011). These data thus contain valuable information on the reionization process that are inaccessible with other, complementary observational probes of the high redshift Universe.
Existing spectra of the brightest known quasars at z ≃ 6 (zAB ∼ 19.5) typically have moderate resolution (R ∼ 3000 − 6000) and/or S/N ∼ 20, even when using the largest of the current generation of optical telescopes (Fan, et al., 2006). Moreover, these spectra are attainable from a subset of known z ∼ 6 quasars only. This lends these data limited power for distinguishing among differing reionization histories due to cosmic variance uncertainties arising from the inhomogeneous nature of the intervening IGM density and ionization state (Mesinger, 2010). A handful of high resolution spectra (R ∼ 40000) at z > 6 have also been attained at moderate S/N ∼ 10−20 for the very brightest quasars, although these typically require exposure times of texp > 8 hrs with an 8 − 10 m class telescope (Calverley, et al., 2011). The improvement in sensitivity provided by the GMT, combined with high resolution optical spectroscopy with G- CLEF, will thus provide the capability to probe the IGM toward the tail-end of the cosmic dawn era from z ≃ 5 − 6.8 with high spectral resolution and superior S/N. Furthermore, larger data sets will also be possible with access (at lower S/N) to high resolution data for fainter background quasars (zAB ∼ 21.0, e.g. Willott, et al., 2010), and possibly GRBs, resulting in a commensurate improvement in the statistical power of the data. This aspect is vital for probing reionization in detail, since it is an inherently spatially and temporally inhomogeneous process.
Detecting high-redshifted Lyman-alpha absorption in z=6-7 quasars: High resolution constraints on the IGM ionization state
As a quantitative example, Figure 1 shows a numerical simulation of intergalactic Lyα absorption in the spectrum of a z = 6.30 quasar. The upper panel displays the quasar spectrum at a spectral resolution (R ∼ 3000) and S/N = 20 per pixel similar to data obtained with an exposure time, texp = 1 hr, with Keck/ESI on the brightest (zAB ∼ 19.5) known quasars at high redshift (Fan et al., 2006). The lower panel displays the same spectrum with a resolution and S/N representative of future data obtained with GMT/G-CLEF (R ∼ 40000) and texp = 4 hr. The greater wealth of information available at high spectral resolution enables detailed insight into reionization the physical state of the IGM, facilitating tighter constraints on the ionization state of the IGM and the measurement of the temperature evolution of the IGM.
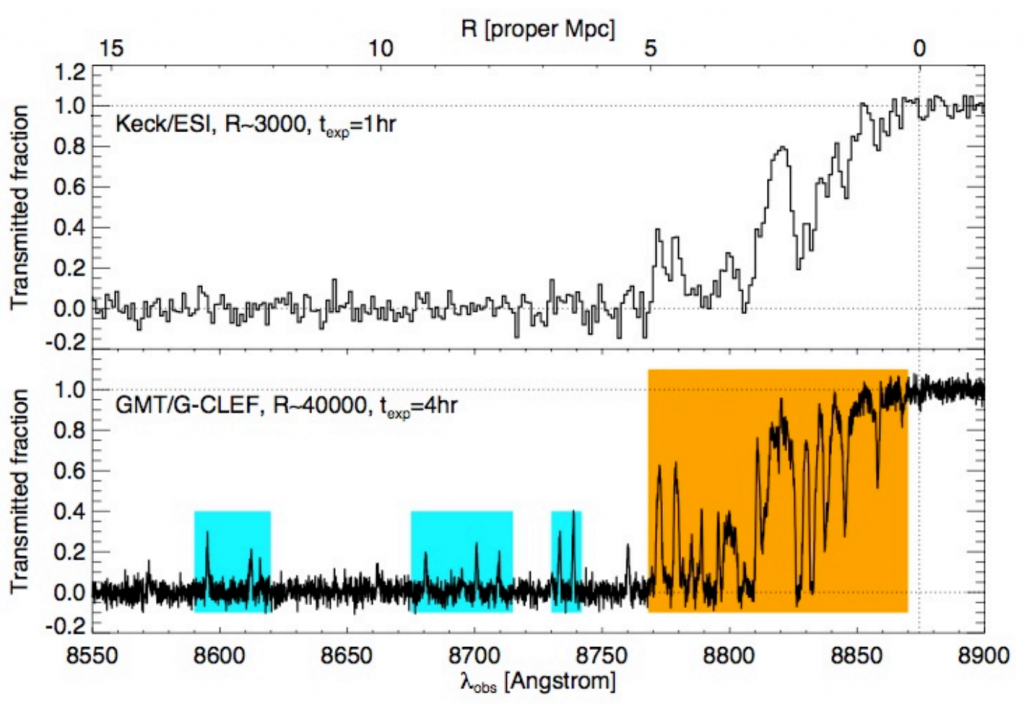
Characterizing the thermal evolution of the IGM following reionization with G-CLEF
The high spectral resolution afforded by G-CLEF also facilitates the measurement of the intrinsic widths of Lyα absorption features in the quasar near-zone (Figure 1, orange shading). Resolving the relatively narrow thermal widths of these lines, b = 12.9 km s−1(T/104 K)1/2, requires high resolution (R ∼ 40 000) spectroscopy. This enables the temperature of the gas in the near zones to be directly measured. Initial attempts at this measurement using high resolution, moderate to S/N∼ 20 Keck/HIRES spectra at z ≃ 6 suggests this approach has promise (Bolton, et al., 2012); larger data sets and higher signal-to-noise will be in reach with GMT/G-CLEF. The ability of the G-CLEF to obtain such high quality data will provide a valuable step forward in our understanding of the physical state of the IGM approaching the cosmic dawn epoch at z > 5.
A Search for Variations in Fundamental Constants Over Cosmological Time Scales
The Standard Model of particle physics is arguably humanity’s most successful theory. Its predictions are regularly tested to exquisite precision in laboratories around the world and, so far, experiment continues to agrees with theory But at its most fundamental level the Standard Model seems incomplete: it is parameterized by several dimensionless “fundamental constants”, such as coupling constants and mass ratios, whose values are not predicted by the Model itself. Nor can the Standard Model say anything about their constancy. Given that experiments done on Earth have only happened at a particular time and location in history, there’s the possibility that these constants may have different values at different epochs and regions in the Universe. Therefore, it is imperative to measure the constants in widely separated regions of the distant Universe and throughout its history.
The most precise cosmological probes for measuring α are absorption lines arising in gas clouds which happen to intersect the sight-lines to bright background quasars. It has been shown, albeit with large systemmatic errors, that the value of the fine-structure constant, α ≡ e2/ħc, is different from the value determined in laboritories when measured at various redshifts (Murphy et al. 2003; Murphy et al. 2004) and locations (Webb et al., 2011; King et al., 2012).
Efforts to refine these measurements continue, but they are united by an important weakness: they rely on quasar spectra obtained with fairly unstable slit spectrographs and are calibrated using separately-observed arc lamp exposures. The fundamental physical question at hand demands much more accurate and robust wavelength calibration of quasar spectra. And if the spatial variation claims are to be confidently ruled out (or confirmed!), a statistically meaningful sample, spread over (some of) the sky, is required together with much tighter constraints on α-variation in individual absorption systems
Determining the constancy of α over cosmic time with G-CLEF
Addressing the three main challenges specified above requires a sample of at least ~20 QSOs, spread over reasonable redshift, RA and Dec. ranges, with each absorber providing a statistical precision of ⩽1ppm per absorber. The statistical precision achievable on α-variation is driven mainly by the spectral S/N. The S/N requirement for the above G-CLEF program can be estimated by comparison with the large Keck and VLT absorber samples of Murphy et al. (2004) and King et al. (2012). There, an absorber with a typical number of “many multiplet” transitions (~7) and a S/N of ~30 per 2.5 km/s pixel yielded typical statistical errors of ~10 ppm on ∆α/α. Assuming we can use a similar number of transitions per absorber in a G-CLEF spectrum, and using the HR mode with 1 km/s pixels, the S/N required is 30 × 10ppm/1ppm × 1/√2.5 = 188 per 1 km/sec pixel. This is an upper limit because, in practice, some relatively unblended “clouds” will exist in some absorbers and so the higher resolution of the G-CLEF observations (R=100 k vs. ~50-60 k for Keck or VLT) will increase the precision on ∆α/α.
The Keck and VLT absorber samples of Murphy et al. (2004) and King et al. (2012) also allow a rough prediction of how many bright QSOs contain at least one “useful” absorption system, i.e. one where at least ~7 of the transitions shown in Figure 5.8 strongly absorb the continuum (to optical depth > 0.5). A conservative estimate is that ~30 southern QSOs brighter than mag (AB; rband)=17.0 contain 2 or more such absorbers. The G-CLEF ETC reports that with this AB magnitude, a 3000s exposure provides S/N=32 per 1-km/s pixel in HR mode with default settings (airmass=1.2, lunar phase=7 days, seeing=0.7 arcsec). Assuming a 5-min overhead per exposure, achieving a S/N of 212 would therefore require ~35 hours per QSO. With ~20 QSOs this amounts to ~700 hours, or ~70 nights. Some QSOs will be brighter and less time can be spent on them. Since observing as “faint” as 17.0 mag allows for selecting QSOs with ⩾2 high quality absorbers per sightline, this will yield ⩾40 ∆α/α measurements with a minimum statistical precision of 1ppm per absorber, i.e. an ensemble precision of ~0.16 ppm. This is unprecedented “precision fundamental physics” in the distant Universe. Spread over the sky, these measurements will strongly rule out – or confirm – the current evidence (or any near-future incremental improvement in it) for time and/or spatial variations in α.
Target | NObj | Resol. | S/N | tExp | Goal Result |
---|---|---|---|---|---|
QSO with z ⩾ 2 absorption systems | 20 | 100,000 | 212 | 35 Hours | Many-multiplet determination of constancy of α |